H Proposal Defense
Topic
The Performance of Thick Gaseous Electron Multiplier Preamplifiers (THGEM) as a Neutron Sensitive Detector.
Introduction
What is main point of the paragraph. 1st sentence in paragraph introduces the main point. 2-4 sentences supporting the main point. 3 - 5 summarized the point
I propose to construct and measure the performance of a fission chamber which has been instrumented with preamplifiers known a Thick Gas Electron Multipliers (THGEM). This Fission chamber device is a gas chamber which has a fissionable material inside. The fissionable material will produce fission fragments when an incident neutron of sufficient energy impacts material.
The objective of this work will be to measure the performance of the THGEM, and then its performance as it is coated with U-238 to detect fast neutrons that produce fission fragments from U-238 within the ionization chamber. This fission chamber-like device will have the advantage of measuring the location of the incident neutrons on the face of the detector.
Gaseous detectors using Gas Electron Multipliers <ref name="Sauli1997">F. Sauli, et al, NIM A386, (1997) 531-534 </ref > are becoming common in nuclear and high energy physics experiments. The Gas Electron Multiplier acts as a preamplifier inside the detector allowing the detector's charge collection elements to decrease in size. A kapton-based gas electron multiplier (GEM) requires the technology to etch an array of holes in thin foils.
Thick Gas Electron Multipliers (THGEMs) preamlifiers have the same aim, but it is designed in a millimetre scale. THGEM may have 2 mm diameter holes manufactured using a CNC machine using a foil has a thickness of 0.5-1mm.
The gas electron multiplier detectors are supported by a read out wire net to get the signal; the read out wire net is connected to a gum stick and a break board then to the daq system. A software (coda) is responsible for collecting and filing the data, then analysing the data by means of ROOT (software package).
Neutrons are nucleons with a neutral charge; they interact generally with high atomic number elements that have high cross sections for certain reactions. Mostly, the neutrons interact with matter by fission, capture, conversion (to an alpha or a proton) and scattering.
The quality of the detected signal is dependent on many factors. Like the thickness of the coated radioactive material for U-238 or Th-232, the detector efficiency when it is coated by different thicknesses of the neutron sensitive material, Also the design for the THGEM card and the gain obtained with the least sparking rate under a voltage difference to the top and bottom of each THGEM card.
Chapter 1
Electron Multiplication (Least fission fragment K.E needed for ionization)
Electrons are fermions, negatively charged particles, usually bounded to the nucleus of an atom. Ionization is the process by which electrons are liberated from the confines of an atom. The minimum amount of energy required to liberate the electron is referred to as the ionization energy; when the electron becomes free moving with a certain velocity, then the energy transferred to it is greater than the ionization energy. The passage of heavy ions through a gas is one way in which to transfer energy to the bound atoms of a gas and liberate their electrons.
Least fission fragment K.E needed for ionization
For a positively charged particle (like a fission fragment) with a certain kinetic energy in an argon gas, electrons will start scattering around the path in all directions because of bremsstrahlung radiation produced by the decelerating by the charged particle, the charge particle is colliding directly with the gas atom, or the primary electrons are producing secondary electrons by electron-electron interactions.
In the case of low interacting particles with the gas, so the number of the electrons released will not be enough to give an indication of the existence of these particles, so any electron produced primarily should have a larger multiplication.
The THGEM card is designed to have a high voltage difference between the top and the bottom side; an electric field will appear with its lines more concentrated in the drilled holes. As free electrons are produced, they are going to be collected by the surrounding electric field toward the card holes. As the electron passes through one of the holes, it will be multiplied, then the multiplied electrons may get multiplied again if the detector is supported with an additional one or more THGEM cards. <ref name="Sauli1997">F. Sauli, et al, NIM A386, (1997) 531-534 </ref >
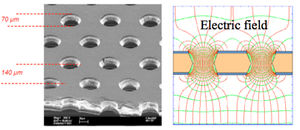
Microscopically, the electron is accelerated when it passes through a hole in which the electric field lines diverge strongly. The electron increased kinetic energy becomes sufficient to ionize other electrons bound to the gaseous atoms. Electrons from this secondary ionization are then accelerated and become the initiators of further ionization events. This process is known as Gas Avalanche Multiplication. <ref name="Shalem2005"> Shalem Chen Ken, "R&D of a novel gas electron multiplier – the THGEM", Master Thesis,the Scientific Council of the Weizmann Institute of Science </ref >
Gas Electron Multiplier (GEM)
The Gas Electron Multiplier (GEM) was invented by Sauli<ref name="Sauli1997"/> in 1997 using recent developments in lithography. The device is made from "flex circuit" technology which is based on 50 micron thick kapton foils clad on both sides with 5 microns of copper. Lithography is used to etch a staggered pattern of micron diameter holes equally spaced by distances comparable to the hole diameter. The small size facilitates the use of low voltages (300 Volts) in order to generate the electric field for amplification. By comparison, the typical drift chamber, operating on the same principle, would need more than 1 kV to establish a similar electric field. The GEM foil is flexible enough to be curved, allowing cylindrically shaped ionization chambers with larger active areas.
GEM has a micrometer scale for the design that makes it hard to maintain. Its sparking rate increases rapidly as the voltage increases from 525V to 600V regardless of the pattern geometry, <ref name="A.Bressan1999">A.Bressan, et al, NIM A 424 (1999) 321—342</ref > but it has a low level noise since the operating voltage around 350V.
When the GEM card is designed in millimeter scale; the new design is called THGEM (thick gaseous electron multiplier)card. With the new design for THGEM, the foil becomes robust with a higher operating voltage and relatively higher gain, but the detector may have a higher noise that can overwhelm the detector signal.
Chapter 2
Detector Structure and Geometry of Thick Gaseous Electron Multiplier (THGEM)
The THGEM detector consists of a gas chamber, THGEM cards, a charge collector composed of strips, and a high voltage distribution circuit. A THGEM card is made from FR4/G10 clad on both sides with copper. The THGEM card is a 12x12 cm square plate that is 1 mm thick with 17 um thick copper cladding.<ref name="G. Agócs"> G. Agócs,JINST 3 P02012 2008</ref> Each card is chemically etched to leave a copper trace around the perimeter of the card that is 10 cm on each side as shown in the figure XX. A thin layer (5 microns) of resistive paste (ED-7100) is applied to the card to permit current to flow on the surface. The card is then machined to have a 0.5 mm diameter hole. The resistance paste near the hole is machined away to form a 0.2 mm diameter rim around the hole. The holes are formed in a staggered array with a pitch of 0.8 mm.
THGEM cards are surrounded by a chamber made of two pieces of machined ertalyte (plastic) ; with a kapton window on the upper piece as shown in the figure. The two plastic parts are collected together by a number of M3 plastic screws located around the detector window to form a cavity to have the cards in an insulated environment from the surrounding atmosphere. A 90/10 percent Ar/CO2 gas is used to generate electrons as the ionizing particles pass through it.
The THGEM cards are fixed in place using holders and separators in each corner to have transparency through the holes, separated from each other by a vertical distance of approximately 2.6 mm. A drift voltage card made of copper paper is placed at the top of the cards with a distance of 2.6 - 4 mm from the last THGEM card.
A read out plate exists before the first THGEM card with a separation distance of 0.5 mm. The read out palte is connected to 16 connectors, each connector has 20 traces. The connectors are then connected to a 130 pin connector by using a VFAT card which is also connected to a break board to give a digital signal from the detector.
Chapter 3
The Detector Physical Properties
Ionization and Calorimetry
Ionization rate is the number of the electrons released from the gas atoms in a certain period of time. Generally, the amount of the energy needed to have an ionization event in a gas is on average the same, regardless of the incident particle type or energy as shown in the following table in case of argon gas.
Type of particle and its energy | 9 keV x-rays | 10 keV electrons | 40 keV electrons | x-rays Ar-37(K-capture)(5-25 keV) + beta | alpha 7.68 MeV | 340 MeV protons |
Energy per ion-electron pair (eV) | 27.9 +_ 1.5 | 27.3 | 25.4 | 27.0 +_ 0.5 | 26.25 | 25.5 |
Fission fragments are also source of ionization when they penetrate an argon gas chamber, the energy used for such an event is highly dependent on the fission fragment mass and velocity. When a fragment has low velocity, recoiling is competitive process to decrease the probability of ionization. In the case of a high velocity fragment, the probability of ionizing the gas increases to be closer to that of the alpha particles as they are penetrating the same medium.
In more specific studies that are based on classifying the fission fragments into light and heavy fission fragments, they tried to measure \delta E (ionization defect), the degree to which the total ionization fails to give a true measure of the particle energy.As a high velocity particle passes through the argon gas, \delta E is 780 keV. Fission fragments' passage through the argon gas gives \delta E of 2.5 MeV for light fission fragments(of energy 98 MeV), and 4.2 MeV for heavy ones (of energy 67 MeV).
Those measurements are not an absolute evidence concerning the ionization of the fission fragments, but they give an idea for researchers who are working in collecting calorimetric or mass spectrographic data. <ref name="Valentine">J.M. valentine, C.Curran, Reports on progress in physics,21, 1 (1958) 1-29 </ref >
Signal Size
The signal of the detector appears as a result of the electron multiplication, so the effect of the ions and fission fragments on the signal is negligible. The previous result can be proved mathematically or by the simulation.
Not only do the free electrons share in the signal, but the electronics used to detect the signal do as well. The figure shows a fast single photon pulse combined with two noise peaks caused by the electronics. <ref name="Chenchik2004"> R.Chechik,A.Breskin,C.Shalem, NIM A535, (2004) 303-308 </ref >
Furthermore, the signal reflects the magnitude of gain obtained as shown in the figure which represents a 60 keV X-ray signal when the gain magnitude is 180 and 220 respectively.<ref name="Bondar"> Bondar,A.Buzulutskov,A.Grebernuk, aXiv:0805.2018 </ref >
The expected signal from the designed detector is composed of electron multiplication peak, background peak(s) of the radioactive isotope used (even U-38 or Th-232) and electronics noise peaks.
Radiation Backgound for Th-232 and U-238 Decay
The background energy spectrum for Th-232 has a main peak for alpha decay of energy 4082.8(14) keV combined with gamma rays of energy 63.81(1) keV and intensity 26.3(13) percent.<ref name="Th-232"> http://home.fnal.gov/~hannahnp/decay/decay.html, Jan.5 2011,http://atom.kaeri.re.kr/cgi-bin/nuclide?nuc=Th-232</ref >.
U-238 has also a close background spectrum as being mainly an alpha emitter, but decay alpha energy is 4274(5) keV with less gamma
intensity 1.02(15) percent when its energy is 113.5(1) keV.<ref name="U-238"> Fermilab,http://home.fnal.gov/~hannahnp/decay/decay.html, Jan.5 2011,http://home.fnal.gov/~hannahnp/decay/U238.html</ref >
![]() .<ref name="Th-232_d"> http://home.fnal.gov/~hannahnp/decay/decay.html, Jan.6 2011,http://atom.kaeri.re.kr/cgi-bin/decay?Th-232%20A </ref > |
![]() .<ref name="U-238_d"> http://home.fnal.gov/~hannahnp/decay/decay.html, Jan.6 2011,http:http://atom.kaeri.re.kr/cgi-bin/decay?U-238%20A </ref > |
The ionization from alpha particles and gamma rays is considered negligible compared to the one for any fission fragment,because of the enormous difference in mass, charge and kinetic energy. But when the fission fragments are absent, there will always be a signal representing the alpha decay.
Neutron fission X-sect for U-238 and Th-232
The cross section is the proportionality constant for the relationship between the particle travelling distance dx and its probability to make an interaction.
The cross section values are represented as a function of energy. The importance of these curves gives the value of the cross section for each energy and shows the resonance peaks. Theoretically, there is not any model that gives a detailed prediction of cross section curve, but statistically it is possible to evaluate the parameters for an assumption that describes part of the cross section curve within a certain error.
Neutron fission is one of the interactions commonly taking place spontaneously or under certain experimental conditions. An incident neutron moving with kinetic energy hits a nucleus to produce new nuclei (fragments) and particles.
Neutrons are classified depending on their kinetic energy into three types: thermal, intermediate, and fast neutrons. The following table shows the range of each type and additional types of neutrons that are important in applications with neutron energy less than the intermediate range.
Type | Energy |
Thermal | 0.025 eV |
Epithermal | 0.025 eV – 0.2 eV |
Resonance | 1 eV – 1000 eV |
Intermediate | 1 keV – 500 keV |
Fast | > 0.5 MeV |
<ref name="Dostal">General principles of neutron activation analysis, J. Dostal and C. Elson,p 28 Figure 2.3.</ref> <ref name="James">7-Ch. Jammes, P. Filliatre, B. Geslot, L. Oriol, F. Berhouet, J-F. Villard, “Research activities in fission chamber modeling in support of the nuclear energy industry”, ANIMMA International Conference, 7-10 June 2009, Marseille, France </ref>
U-238 and Th-232 belong to the actinides. They are characterized with relatively high neutron fission cross section for fast neutrons (specifically for neutron energy higher than 1.5 MeV ). They also have a high elastic cross section in the range that the detector is designed for.
![]() |
Efficiency and Sensitive THGEM
The efficiency of a detector is the ratio of the detected events to the total number of events emitted by a source. The intrinsic efficiency has the same definition but it is independent on the detector geometry, and basically considers the interaction cross section of the incident particle with the detector material.<ref name="William"/>
Efficiency requires maximizing the rate of interactions by an appropriate choice for neutron fission sensitive material and the thickness of the sputtered layer over the THGEM cards. Also increasing ionization rate for the fragments under a certain pressure in a highly dense electric field environment, the last condition demands a thorough study for the voltage applied on THGEM cards and distances between them.
Sensitivity is a scale for the detector's ability to output a usable signal as a result of a particle interaction within the detector.<ref name="James"> Ch. Jammes, P. Filliatre, B. Geslot, L. Oriol, F. Berhouet, J-F. Villard,“Research activities in fission chamber modeling in support of the nuclear energy industry”, ANIMMA International Conference, 7-10, June 2009, Marseille, France.</ref>
The sensitivity of THGEM as a neutron detector mainly depends on neutron energy range, material cross sections for ionization, neutrons rates of interactions, detector mass, background, noise (sparking and electronic devices used to collect the signal), materials surrounding the detectors and the strength of the electric field.<ref name="James"/> <ref name="William">William R. Leo,Techniques for nuclear and particle physics experiments,1st edition,Springer Verlag, 1995.</ref>
THGEM detector is going to give a digital signal, coming from a defined localized point on the read out plate. This will give information about the real sensitive area of detector to the detected electrons which will be considered a reference for a new size of the future detectors used for the same purpose.
Furthermore, the detected signal will show the energy(s) where the detector is going to be the most sensitive if a calibration curve is available.
Expectations
THGEM as a neutron detector physical properties will be investigated. A clear experimental procedure to get the maximum gain with the least sparking through the detection process. Also, a comparison between the detector efficiency using Th-232 and U-238 and the effect of thickness of coated material on the efficiency. Finally, recommendations will be suggested to improve the next neutron detector design.
References
<references/>
Paul Reuss ,Neutron physics,L'editeur EDP Sciences,2008.