|
|
Line 38: |
Line 38: |
| Table : energy range of interactions for photon neutron and proton. | | Table : energy range of interactions for photon neutron and proton. |
| | | |
− | There are several widely accepted Monte Carlo codes for radiation transport simulation. Some are well-documented and commonly used, others less well so. We are planning to use two such codes should the NNSA Center of Excellence be established: MCNPX and GEANT4.
| + | A user has a great versatility in defining sources and materials, turning on and off different physics processes and choosing tallies to be performed. |
− | | |
− | MCNPX is an acronym for Monte Carlo N-Particle EXtended. MCNPX can be used for neutrons, photons, protons, electrons, alpha particles, and many more. It is supported on most of the available operating systems, including Microsoft Windows, Mac OS, Linux, and many other UNIX-like operating systems. It can also be compiled for multi-processing through message-passing interface (MPI). MCNPX graphic routines are supported through numerous plotting packages.
| |
− | | |
− | MCNPX can be used in many fields of study where knowledge of how radiation interacts with matter is necessary. Common examples include nuclear fuel cycle research, nuclear criticality calculations, medical physics and radiography, isotope production, and detector development – an area we are planning to pursue mostly. MCNPX tracks neutrons from 0 to 20 MeV for all isotopes, and up to 150 MeV for some isotopes. Photons are tracked from 1 keV to 1 GeV, and electrons from 1 keV to 1 GeV. The energy range of the above particles can be extended by using physical cross-section models. Transport capabilities of many other particles can also be extended using different models, such as Bertini or ISABEL.
| |
− | | |
− | The code treats an arbitrary 3-D configuration of materials in geometric cells bounded by first and second degree surfaces, such as planes, spheres, cylinders. Point-wise cross-section data is used. For neutrons, all reactions given in particular cross-section evaluation, most recently, ENDF/B-VII, are accounted for in the transport, and the user may select which cross-section evaluation to use. Thermal neutrons are described by either the free gas or S(α,β) model. For photons, the code accounts for incoherent and coherent scattering, the possibility of fluorescent emission after photoelectric absorption, and absorption in electron-positron pair production. Electron/Positron transport processes account for angular deflection through multiple Coulomb scattering, collisional energy loss, with optional straggling, and the production of secondary particles, including K-shell X-rays, knock-on, and Auger electrons, Bremsstrahlung, and annihilation gamma rays from positron annihilation at rest. The code does not include the effects of external or self-induced electromagnetic fields. Photonuclear physics is available for a limited number of isotopes. The source definition capabilities of MCNPX allow users to specify almost any source. Source variables may be discreet, or may be specified as distributions. Additionally, source variables may be defined to be functions of other source variables, providing the user great versatility in defining sources.
| |
− | | |
− | MCNPX offers a significant quantity of built-in Monte Carlo variance reduction techniques. Important splitting and rouletting can be performed, if the user specifies splitting parameters for the geometry. Additionally, space-energy or space-time dependent splitting can be performed using the Weight-Window Technique, a weigh-dependent splitting reduction technique. Time, energy, and weight cutoffs are also included in the MCNPX code.
| |
− | | |
− | The tallying capabilities are extensive. Some of the basic tallies include surface flux and current tallies, volume flux tallies, point and ring detectors, heating tallies, and pulse-height tallies to simulate radiation detector responses. The tallies can be performed over many individual cells or surfaces, as well as the combination of cells of surfaces and can be binned by energy, time, direction, special multipliers, such as cross-section surface and cell flagging, and user-specified bins. MCNPX also has the capability to perform mesh tallies over a user specified mesh, independent of the geometry, and which can also be binned by energy. MCNPX offers a significant quantity of platform-independent plotting capability. Geometries can be plotted and insured accurate through the variety of the geometry plotters. Tally results and cross-sections can be viewed through MCNPX’s tally and cross-section plotting. The results of mesh tallies can also be visualized with a tally plotter.
| |
Revision as of 00:33, 5 March 2012
They will support "development of advanced simulations and measurement techniques leading to improved radiation and particle detection methods in terms of energy, temporal and spatial resolution".
GEANT and MCNPX are two simulation packages commonly used by researchers in Nuclear physics to develop experiments and interpret experimental resuls. While GEANT4 is a publicly available open source package, MCNPX requires a license and may only be available as an executable to those who are not elibigle for a source license. GEANT and MCNPX have substantial overlap in the models and data they employ to predict how particles interact with matter. For example, both utilize the Evaluated Nuclear Data FIle (ENDF) from the National Nuclear Data Center as input to their simulation programs.
Historically, MCNPX has been a more complete resource for simulation nuclear reactions than GEANT. Recently however, GEANT4 has been gaining ground and becoming a testbed for such models as the Bertini Cascade model (INCL/ABLA). Although each has their stengths and weaknesses,
nuclear physics students who desire to develop a simulation program, but are not eligible for the MCNPX source code, rely on GEANT4. As a result, GEANT4 has a large development community and more readily used as an education tool for universities who train international students as well as
US students for entry into the US nuclear workforce.
Students working at the Idaho Accelerator Center have a long history of using MCNPX to interpret their experimental results. A recent effort has been made to begin using simulation tools in the design of experiments. Figure 1a is an example of using MCNPX to determine the neutron flux from a proposed accelerator based neutron source. Students in the center have been using GEANT4 to develop an efficient fission chamber. Figure 1b is an example of one student comparing the U-238 neutron induced fission spectrum predicted by the ABLA model in GEANT4 to the IAEA's data evaluation. As an NNSA center of excellence, students will be given the opportunity to contribute to the development of simulations by contrasting their predictions with experimental results.
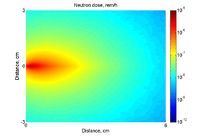 |
|
a Neutron flux mapping for a tungsten cylindrical converter |
b Comparing the U-238 neutron induced fission spectrum predicted by the ABLA model in GEANT4 to the IAEA's data evaluation
|
INTERNATIONAL EVALUATION OF NEUTRON CROSS-SECTION STANDARDS, INTERNATIONAL ATOMIC ENERGY AGENCY,VIENNA, 2007 File:U238-xsection.pdf
absolute f_xsection in the table 7.1 p.91
Data set # 646 pr 648
646: Li Jingwen et al,INDC(CPR)-009 (1986) 7
648:R.K. Smith et al. (1956),Personal communication, G. Hanson (1975)
Table : energy range of interactions for photon neutron and proton.
A user has a great versatility in defining sources and materials, turning on and off different physics processes and choosing tallies to be performed.